Frequently Asked Questions
What are meteorites?
Meteorites are rocks that have been injected as meter-sized objects
into the Earth's orbit following collisions on their parent
bodies. Most come from asteroids; More than 200 come from the Moon and
about 150 are thought to come from Mars.
Meteorites are of
great scientific importance. Some have preserved the record of
processes that occurred before and during the formation of the
planets. This is apparent from the great age of most meteorites: 600
million years older than the oldest known Earth rock, and 500 million
years older than nearly all lunar rocks. The study of meteorites
provides important information about the origin of the Solar System
and the formation of the planets.
Martian meteorites provide
clues about the evolution of this planet that cannot otherwise be
obtained until very expensive sample-return missions are completed in
future decades. Most lunar meteorites sample regions on the Moon that
were not visited during the Apollo missions.
Small rocky
objects in interplanetary space are called meteoroids. When
meteoroids enter the Earth's atmosphere at great speed, they produce
fireballs that can be brighter than the full Moon. During the first
part of their flight through the atmosphere, air friction melts the
surface layer of the meteoroid and raises its temperature to
incandescence. The light phenomenon produced by the passage of a
meteoroid through the atmosphere is known as a meteor. The
brighter meteors made by meteoroids with masses >100 grams are called
fireballs. If a meteoroid is still moving above the speed of sound
after it falls below a height of about 55 kilometers it will create
sonic booms.
Most stony meteoroids undergo fragmentation and
produce showers of glowing objects. Air friction removes the melt from
their surface as it forms, exposing new surfaces that also
melt. Meteoroids can lose more than 95% of their mass during
atmospheric passage.
By the time meteoroids have fallen to
a height of about 20 kilometers, their velocities have been reduced to
about the speed of sound and the meteoroids stop glowing. The final
surface melt on these objects solidifies to form a fusion
crust.
Throughout atmospheric passage, the interiors of
the meteoroids retain their original temperature (near the freezing
point of ice); the internal structure and composition of these rocks
are unaffected by the melting of the rocks’ surface. When meteoroids
reach the Earth, they are called meteorites. The surface of
freshly fallen meteorites might be warm to the touch, but they are
never hot enough to ignite fires when they land.
Meteorites are divided into two main classes: primitive and differentiated
Primitive meteorites
The most abundant meteorites are the chondrites; they comprise
about 90% of the meteorites that fall. Most of these rocks have been
altered by thermal or aqueous processes. Those that have largely
avoided alteration consist of minerals and mineral assemblages (such
as chondrules) produced in the Solar Nebula, the cloud of gas
and dust that formed in the planetary region at the same time as the
Sun. The processes by which these particles were collected and
compacted to form the chondrites are still poorly understood, but it
is clear that these primitive objects experienced little chemical
change in their early history.
Eventually, much larger
objects were produced from these primitive chondritic planetesimals
("infinitesimal planets"). Thus it is reasonable to call the
chondrites the building blocks of the planets.
Chondrites
show a wide range in properties indicating formation at different
places and times (for example, some have high (~20 weight %) metal
contents; others are metal free). Metal-free chondrites contain
similar amounts of iron and nickel as metal-rich chondrites, but these
elements are present solely as oxides or sulfides. The most primitive
chondrites consist of minor phases with a wide variety of chemical
properties; the millimeter-size grains of these rocks formed one at a
time and were never in equilibrium with each other.
[Figure A]. These millimeter-sized objects formed during brief melting
events in the Solar Nebula. The melting events were very short, with
durations of seconds. The physical process that created them is not
known; shock waves and lightning are commonly discussed
possibilities.
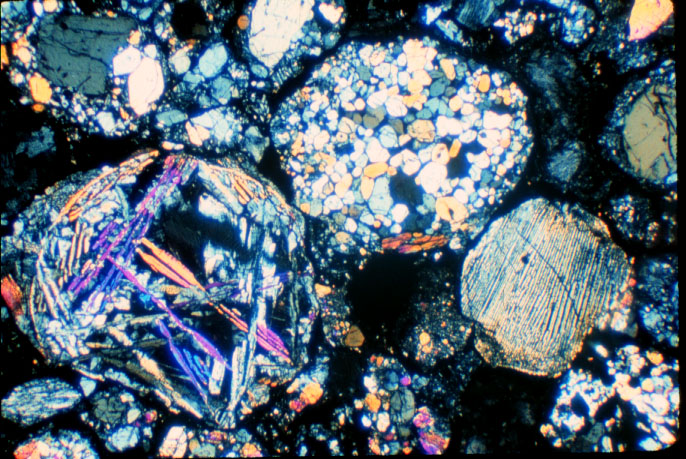
A characteristic of chondrites is their content of chondrules
Figure A
Three complete
chondrules about 1 mm in diameter are visible in this
transmitted-light photo of the Tieschitz chondrite. The colored
minerals are mainly olivine and vary appreciably in shape and size
from chondrule to chondrule. Width of image about 4 mm.
Differentiated meteorites
The differentiated meteorites were formed by melting and the separation of phases
("differentiation"). Heating metal-rich chondrites to sufficiently
high temperatures causes them to melt; immiscible metal and silicate
liquids are produced. The denser metallic melt then moves downward
under buoyancy forces and the silicate melt moves upwards. This is
the main way that iron meteorites and differentiated
stones (basalts such as the eucrites and howardites) formed. The
more refractory silicates did not melt but remained as the "mantle" of
the parent asteroid; the main mantle mineral was olivine (the same
phase as the semi-precious gem peridot).
The iron
meteorites consist of iron-nickel metal together with some other
phases. Many iron meteorites show a Widmanstätten or octahedral
pattern such as exhibited by polished and etched samples of the
Buenaventura iron [Figure B].
The light phase that forms bands
oriented in four directions is a low-nickel (about 6% nickel) alloy of
iron. This section of Buenaventura also includes some irregular
patches of schreibersite, an iron-nickel phosphide mineral.
The most common differentiated stones are the basalts; they are similar in composition to lavas extruded by volcanoes on Earth. The texture of the Pasamonte eucrite is typical of those produced in rapidly cooled lavas [Figure C]. This meteorite created a long dusty tail in the atmosphere when it fell in New Mexico in 1933.
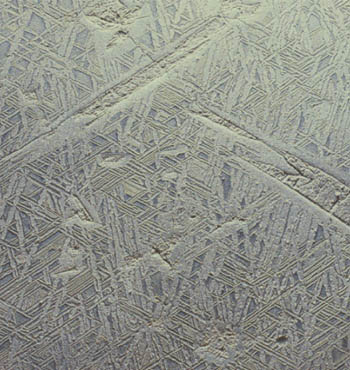
Figure B
Close-up view of the Widmanstätten pattern of the Buenaventura IIIAB iron meteorite. The pattern is an intergrowth of two different iron-nickel minerals (kamacite and taenite) that contain different amounts of nickel. The dominant pattern is formed by the low-nickel phase kamacite (the light-colored bands). Note that there are thin bands oriented at about 45º and 135º relative to the base of the picture; two other sets of bands are wider and somewhat irregular in shape. Some regions with grainy textures and irregular shapes are iron-nickel phosphides. The meteorite was found in Mexico around 1969. (Photo by G. Natzler)
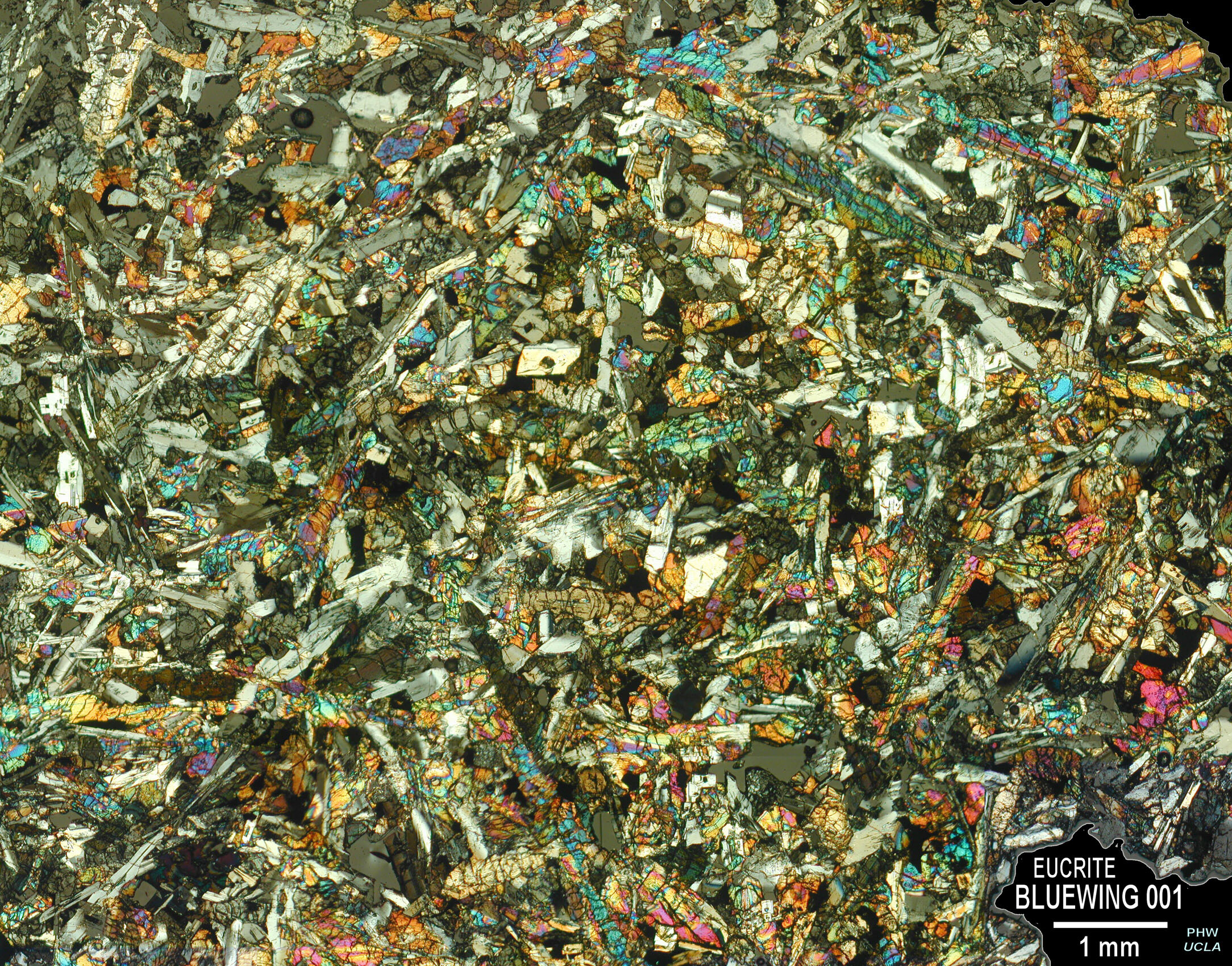
Figure C
This thin-section view of the eucrite Bluewing 001 reveals a basaltic (more specifically “subophitic”) texture, indicative of rapid crystallization of a fast-cooling magma at the surface of an asteroid or planet. The major minerals (pyroxene, various bright colors; plagioclase, shades of grey) form elongated intergrown grains up to a few millimeters in length. This 6.1-g meteorite was found in Nevada in 2000. (Photo by P. Warren)
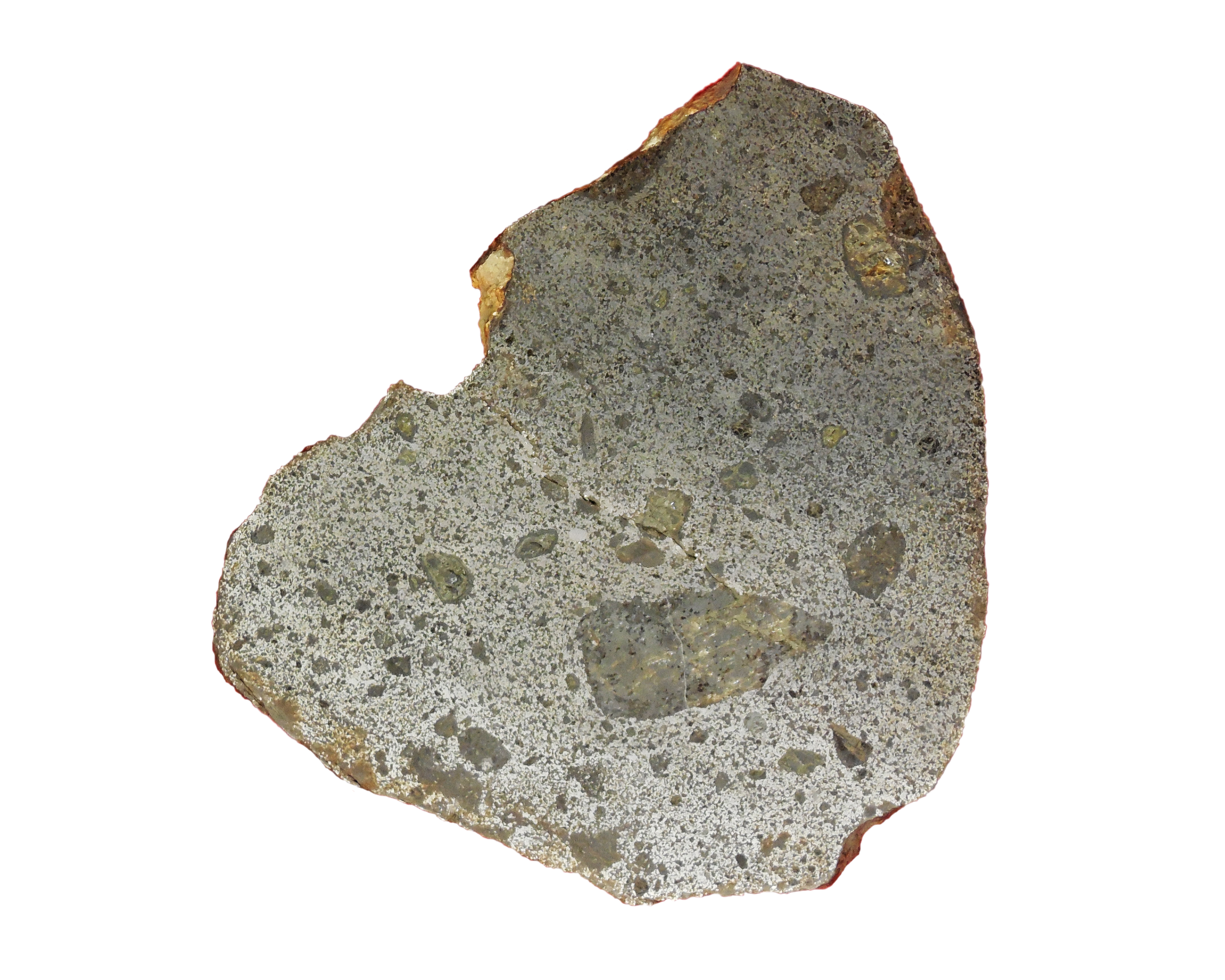
Figure D
The Emery mesosiderite. This stony-iron meteorite was found in South Dakota around 1962. It consists of about 50% iron-nickel metal and 50% silicates including the large triangular clast. It is speculated that these meteorites formed during the collision of an asteroid with a molten core with a second asteroid with basaltic materials on the surface. (Photo by G. Natzler)
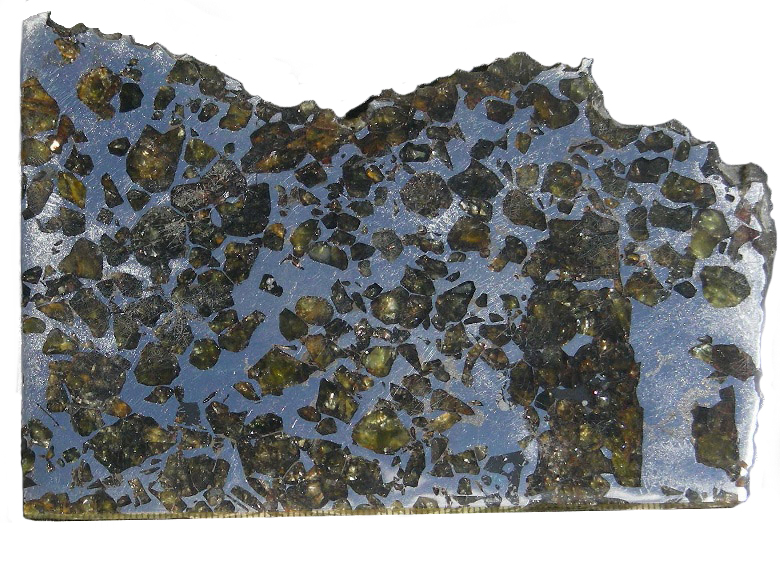
Figure E
The Seymchan pallasite. In Seymchan the yellow silicate olivine grains are 4 to 7 mm across and are embedded in a matrix of nickel-iron metal. These meteorites formed by the mixing liquid metal with solid olivine at the core-mantle interface of an asteroid. The irregular shapes of the olivine indicate formation by crushing, probably during an impact. Length of section about 14 cm. (photo by J. Wasson)
A curious kind of differentiated meteorite is the group of mesosiderites; these rocks consist of a mixture of basalt (which is commonly formed near the surface of a differentiated asteroid or planet), and metal (which commonly forms cores in planetary bodies). Figure D shows a sample of the Emery mesosiderite from the UCLA collection
The members of one class of differentiated meteorite, the pallasites, consist of roughly equal amounts of metal and olivine; they formed at the boundary between the core and the mantle of their differentiated parent asteroid. In Figure E the olivine of the Seymchan pallasite is yellow and the metal is light gray.
How to recognize meteorites?
Properties important for distinguishing meteorites from normal rocks
Many of us like to check out the rocks we see when we take nature walks; we dream that we may someday find a meteorite. Here in southern California a large fraction of public lands is desert where, because vegetation is scarce, rocks are easy to see. Here are some properties that can be used to help identify meteorites.
Size and Density
Meteorites may vary in weight from a less than a gram to many tons, but typical masses of recovered meteorites are in the mass range 100 to 10000 grams; typical lengths are about 3 to 30 cm. Iron meteorites have very high densities (7.4 to 7.9 times that of water) and weigh about three times as much as Earth rocks of similar size; most chondrites weigh about one and a half times as much as comparably-sized earth rocks.
Characteristic Features
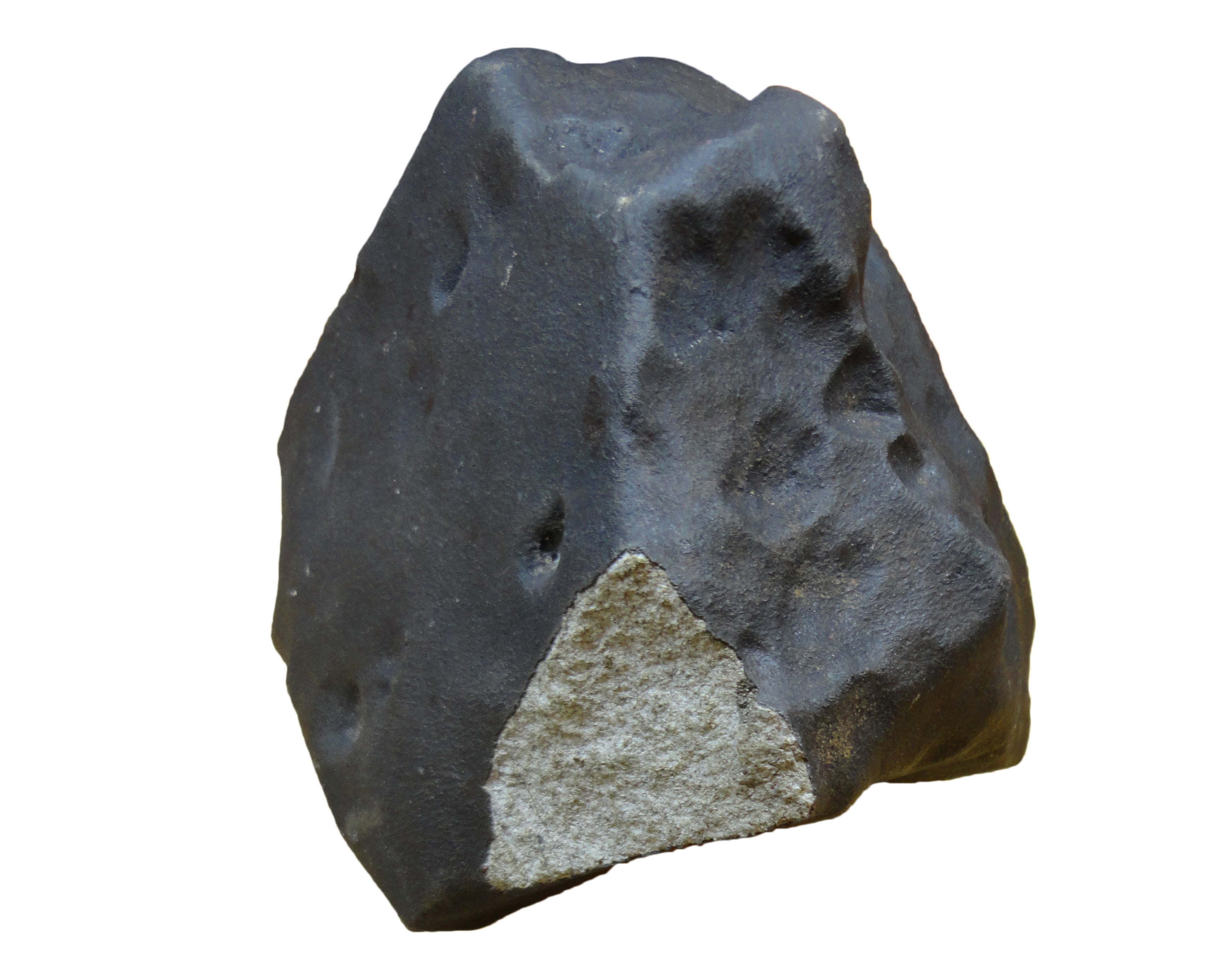
Figure 1
The La Criolla L6 chondrite. This meteorite fell on 6 January 1985 in Argentina. Note how the thin matte black fusion crust contrasts with the light gray interior of the stone. Left-to right length about 18 cm. (Photo by G. Natzler)
Meteorites are generally irregular in shape with rounded edges, but none are completely spherical. The fusion crust of most freshly fallen meteorites is generally black [Figure 1]; on some rare types the crust is light gray. Interiors of most stones, as with the La Criolla chondrite, are generally gray in color.
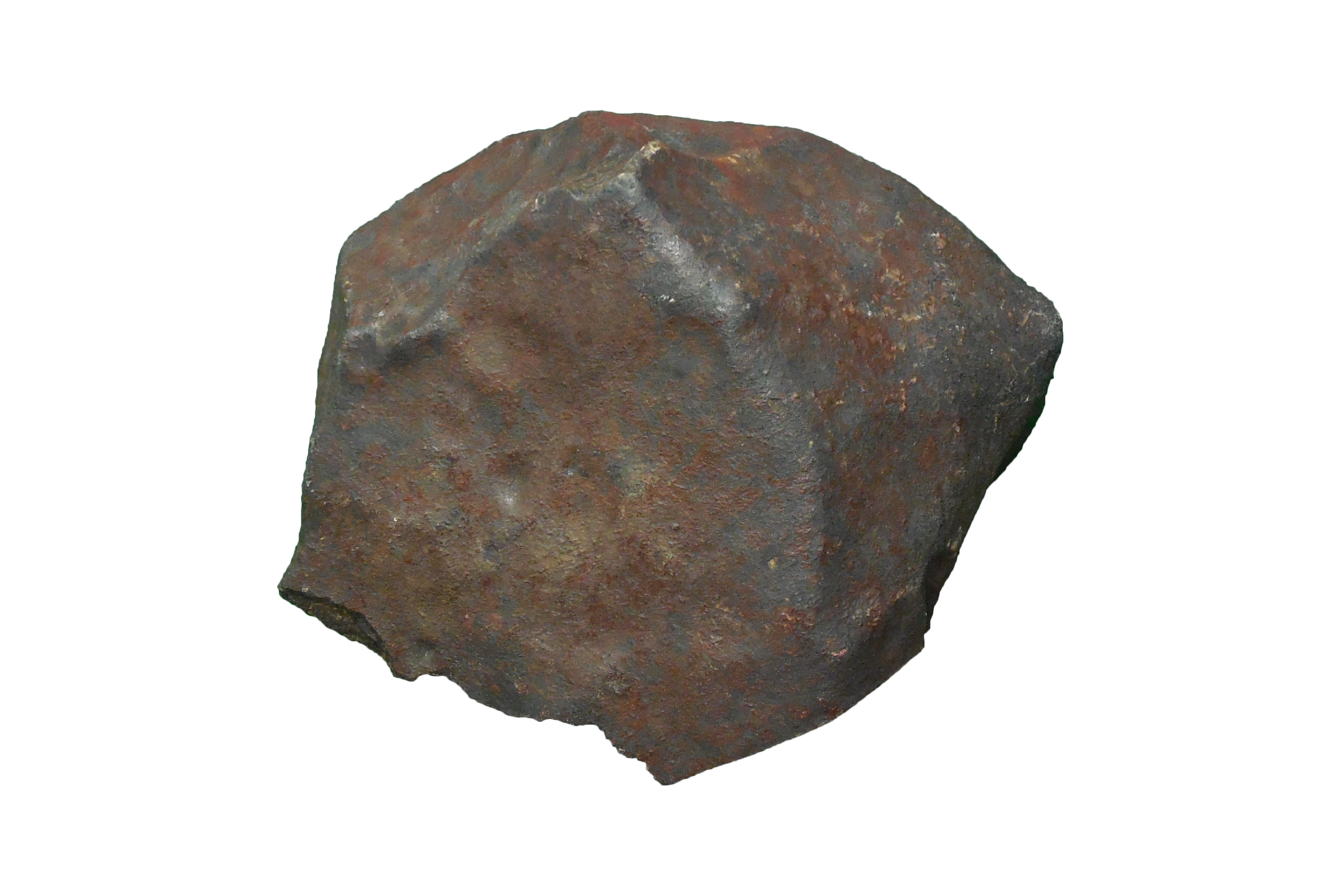
Figure 2
The Ness County L6 chondrite. This meteorite was recovered from a field in Kansas; it has weathered to a mix of brown (iron oxide) and black (original fusion crust). The rounded dimples are typical of chondrite falls. Left-to-right length about 18 cm. (Photo by J. Wasson)
Over the course of decades or centuries, weathering on Earth can change the fusion crust of a meteorite to rusty brown [Figure 2].
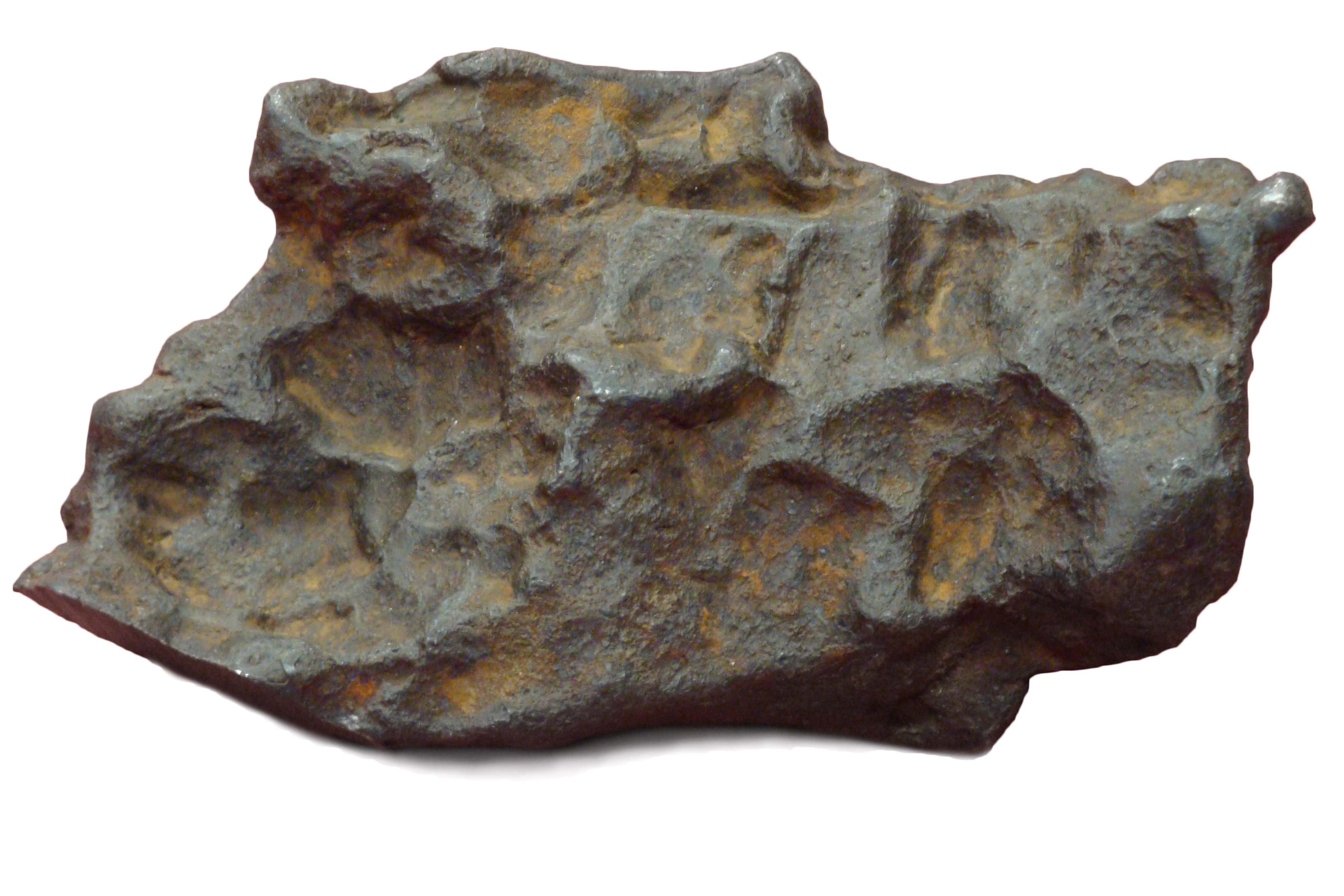
Figure 3
The Kinsella IIIAB iron meteorite weighs about 3.5 kg and is about 20 cm long . This meteorite was found in 1946 in Alberta, Canada. Note the that the depressions on the rusty fusion crust formed during atmospheric passage are deeper than those in the chondrites. (Photo by Gail Natzler)
The smooth surface of the fresh meteorite is often pitted with thumbprint-shaped depressions (called regmaglypts) that are more pronounced on irons than on stones (see the Kinsella iron meteorite [Figure 3].)
No meteorites are porous or hollow.
Tests
a) Shape, color and texture. The first clue that a rock is a possible meteorite is its general shape and color. Freshly fallen stones are black; weathered stones or irons are generally dark brown.b) Magnetism. Because meteoritic metal is always magnetic, it can be attracted with a magnet. Irons are strongly magnetic while most stones will attract a magnet weakly. Weak magnetism can be demonstrated by hanging the magnet on a string, then showing that it swings when the stony sample is passed near to it. But note that not all meteorites are magnetic: lunar meteorites, martian meteorites and some eucrites are essentially metal free.
c) Exposure of the interior. An important test is to grind a corner of the suspected object with an emery wheel, emery stick or silicon-carbide paper. An iron meteorite will show a bright white metallic surface and the vast majority of stony meteorites will show tiny flecks of shiny, silver-colored metal.
If the specimen has a black interior, is highly magnetic but feels less dense than a piece of steel of similar size, it is probably the iron oxide magnetite. Magnetite is the most common field material confused with meteorites, particularly in California. The identification of magnetite can be confirmed by using a streak test. Magnetite gives a black streak on an unpolished (e.g., bottom side of) ceramic tile.
NOTICE: UCLA NO LONGER ACCEPTS SUSPECTED METEORITES FOR IDENTIFICATION.
What to do if you think you have a meteorite?
You may try contacting the Geological Survey of your state, a local college or university or college or a local natural history museum. In addition, there are a few commercial firms that will charge a fee for examining and identifying suspected meteorites.If you see a meteorite fall
In order to assist in the recovery of a recently fallen meteorite, you should note the exact direction in which the fireball disappeared. Select a distant landmark that is in line with the end point of the fireball's path and note carefully your own position. Listen for any accompanying sounds. It is also important to record the time and the brightness, size and shape of the fireball.If you find a meteorite on the ground following an observed fall, take a photograph of it before picking it up. Look around for other specimens; most meteorites break into several pieces before hitting the ground; these pieces could be many tens of meters apart.
You may send this information by e-mail along with any images of recovered meteorites to the UCLA meteorite website: meteorites@ucla.edu